Double emulsion droplets in microfluidic chips: a review
How can we generate double emulsions using microfluidics? How can we control PDMS surface wettability? What are the new microfluidic-based techniques for generating double emulsions? We address these questions in this review.
Introduction to double emulsion droplets production
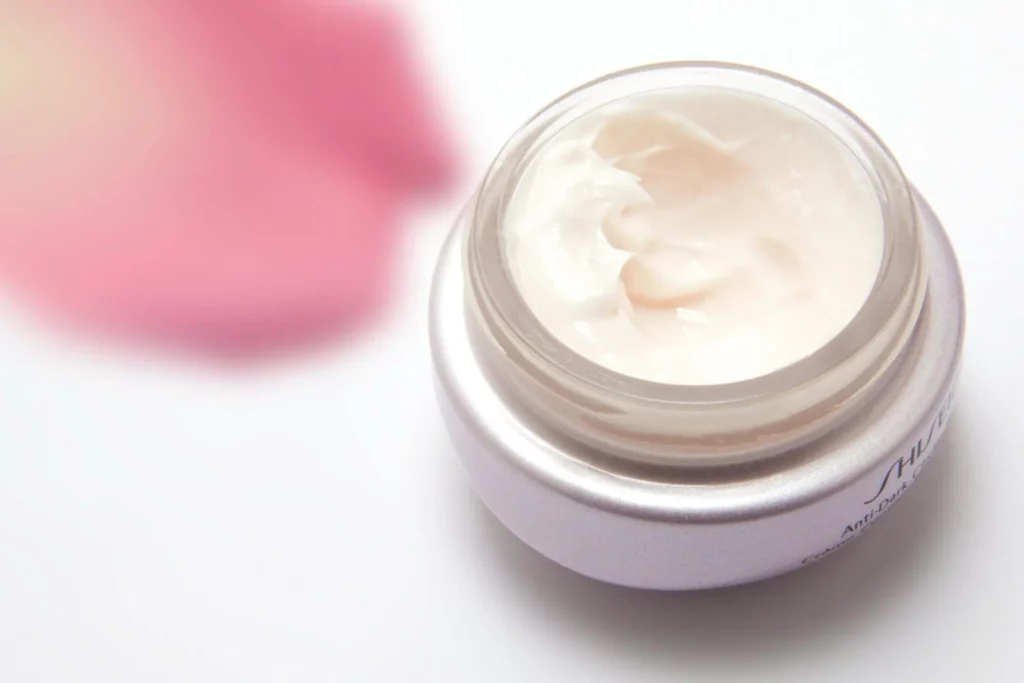
Multiple emulsions are complex polydisperse systems where oil in water and water in oil emulsions exist simultaneously, stabilized by hydrophobic and hydrophilic surfactants. They are promising materials for industrial fields like cosmetics, pharmaceutics, food.
These emulsions in an emulsion can be used to encapsulate fragile compounds (drugs, vitamins, aromas…), protect them during storage, and eventually release them on demand at a suitable time and place.
Typically, double emulsions are made in two steps with two surfactants. For example, to create a water-in-oil-in-water (w/o/w) emulsion, water is first emulsified with an oil phase containing a hydrophobic surfactant and re-emulsified in a second step with a water phase containing a hydrophilic surfactant.
Double emulsion droplets are thus complex to obtain. The second step, in particular, needs to be handled with care to preserve the primary emulsion. In addition, emulsions produced with this technique are polydisperse, which is undesirable for the industry and affects the stability of double emulsions. Microfluidics can address some of these drawbacks and allow the formation of perfectly monodisperse double emulsion droplets in a controlled way.
Microfluidic geometry for double emulsion droplets
There are three significant geometries to generate droplets with microfluidics: flow-focusing, co-flowing, T-junction (Figure 1), glass capillary, and the potential combination of these geometries. These techniques have been adapted for the formation of double emulsion droplets.
The simplest and most widely studied geometry is the succession of two cross junctions, flow-focusing. This configuration is compatible with soft-lithography techniques and can quickly adapt to form high-order multiple emulsions [1].
At the first junction, internal droplets are formed and encapsulated into external droplets at the second junction. Co-flowing configuration is widely used with all fluids flowing coaxially. The fluid that has been dispersed is entirely embraced by the fluid flowing continuously in co-flowing streams, which makes surface treatment unnecessary [2, 3].
Two consecutive T-junctions on glass can produce double emulsion drops with a controlled number of inner drops. This number increases with the increasing flow rate of the internal phase [4]. Glass capillaries are optically transparent, electrically insulated, and chemically robust. Two-step microfluidic emulsification methods were developed in a three-phase glass capillary device, generating high-order multiple emulsions [5]. Combined with co-flow geometry, the microcapillary device can generate monodisperse emulsions in a single step [6].
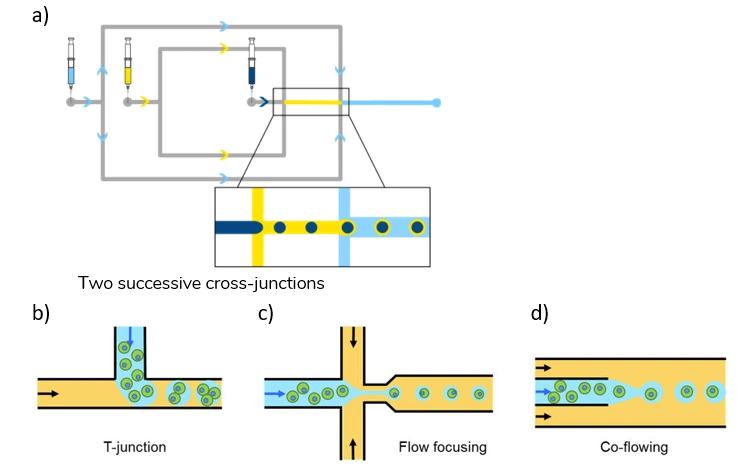
Double emulsion droplets in microfluidics
To generate droplets in a microfluidic chip, the wettability of the walls is a crucial parameter. The formation of double emulsion droplets in a microfluidic chip seems quite complex.
PDMS wettability for microfluidics
PDMS, the most widely used material for microfluidics, is intrinsically hydrophobic, which has consequences for droplet-based microfluidics. To successfully generate droplets, the continuous phase must wet the chip walls effectively. In the case of water-in-oil (w/o) droplets, walls have to be hydrophobic to minimize the contact between the microchannels and the aqueous phase. Therefore, PDMS is ideally suited for the generation of these droplets.Â
Â
However, to generate oil-in-water (o/w) droplets, the continuous phase should not wet the walls; otherwise, droplets would break. Thus, it is not possible to produce o/w droplets with native, untreated PDMS. Moreover, double emulsions (water-in-oil-in-water (w/o/w) and oil-in-water-in-oil (o/w/o)) require more complex, spatially defined control of wettability [8]. Several efforts were made to make PDMS walls hydrophilic and combine PDMS hydrophilic and hydrophobic parts on the same chip [9].
PDMS surface-coating-based treatments
When exposed to oxygen ions generated by plasma cleaner, the PDMS surface is oxidized, and the methyl groups of the surface are replaced by -OH moieties, making it hydrophilic. However, this effect only lasts a few hours because the uncured oligomers in the bulk material will migrate to the surface over time.
Exposure to plasma also activates the PDMS surface: the -OH moieties can create covalent bonds with various silanes, for example [10]. However, it is difficult to coat only a specific part of the microfluidic chip to produce double emulsion droplets.
Hu and colleagues [11], and later on, Abate and colleagues [12] tried coating the device’s walls with a hydrophilic polymer. First, they adsorb a photoinitiator on the walls, and then they inject an acrylic acid solution inside the channels and initiate the polymerization by exposure to UV light. Hu and colleagues worked with benzophenone, whereas Abate and colleagues worked with silanes. Only channels that need to become hydrophilic were coated thanks to a mask during UV exposure.
These techniques are very efficient but also time-consuming. Moreover, injecting liquids into the chips before the formation of double emulsions increases the risk of obstructing the channels.
Bauer and colleagues [13] developed a protocol using layer-by-layer adsorption of hydrophilic polyelectrolytes on the channels, alternating positive and negative charges. The parts that need to be kept hydrophobic are protected by injection of deionized water at a carefully controlled flow rate (Figure 2).
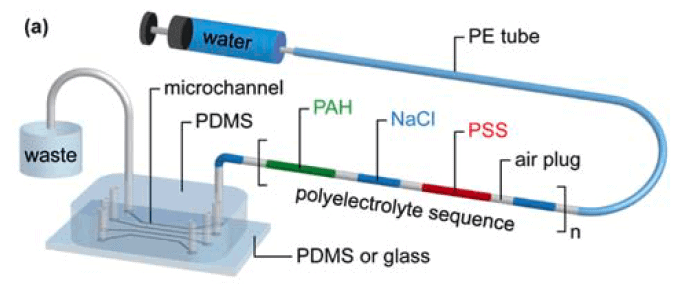
PDMS non-surface-coating-based treatments
More recently, other techniques were developed without any coating. They are based on precise control of the part of the microfluidic chip exposed to plasma.
Kim and colleagues [14] worked with plasma-bonded chips that have recovered their hydrophobic properties. The chips are exposed to plasma again, and oxygen ions enter the chips through the inlets and outlets punched in the PDMS.
PDMS surface is oxidized and turns hydrophilic. Some inlets or outlets are blocked with tape during exposure, and diffusion barriers are integrated into the chip to control the ion’s diffusion spatially. The chip’s geometry must be optimized to allow the targeted channels to be oxidized (Figure 3).
Li and colleagues [15] developed easy and quick techniques to pattern the wettability of the chip by using either epoxy glue or permanent marker. In the first case, glue is removed just before bonding, and in the latter, the marker is rinsed by injecting ethanol into the chip after the bonding. In that way, combining hydrophilic and hydrophobic parts on the same chip is possible. However, since the effect of the plasma is temporary, these chips can be used to produce double emulsions only for a couple of hours before the hydrophobic recovery of PDMS (Figure 3).
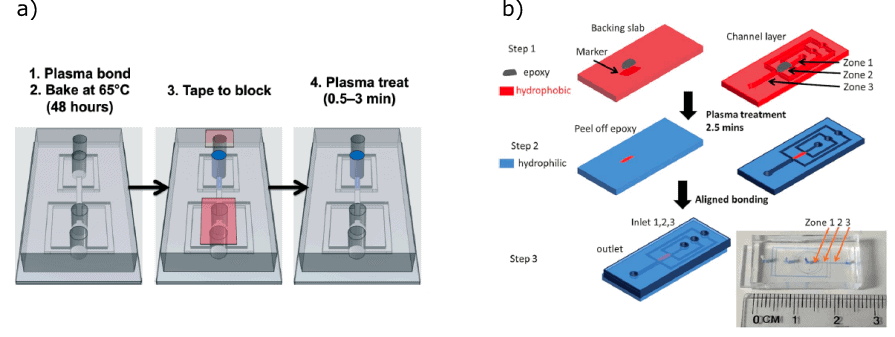
3D-printed microfluidics for double emulsion droplets
Recently, the general concept of emulsion formation has been successfully transferred from PDMS-based to 3D-printed microfluidics [8]. 3D-printed droplet-based microfluidics present unique features such as modular design, facile integration, and parallelization [16] and have demonstrated efficiency for the complicated operation of multiple emulsions [17].
Mannel and colleagues demonstrated a fabrication process in which the first hydrophilic material is 3D-printed onto another hydrophobic resin to print the remaining part of the device.
They changed photopolymer formulations while printing the single layers. O/w droplets are first formed after traveling through the first hydrophobic region and the second hydrophilic region; then, o/w droplets are encapsulated by oil in the third hydrophobic region to form o/w/o double emulsions. This method adopted the planar junction design, though the locally modulated surface wettability led to the stable generation of double emulsion droplets [8, 16].
Ji and colleagues reported pneumatic control of multiple emulsions generation in modular 3D-printed devices consisting of three modules: the function module, T-junction, and co-flow modules. The function module includes a single-inlet, pneumatic control unit (PCU), and dual-inlet modules (Figure 4).
The T-junction module is used for inner droplet generation, and the co-flow module is used for outer droplet generation. Double emulsions with different numbers and compositions of inner droplets have been successfully generated with easy control of the different phases’ flow rates thanks to a microfluidics pressre-driven flow controller [17].
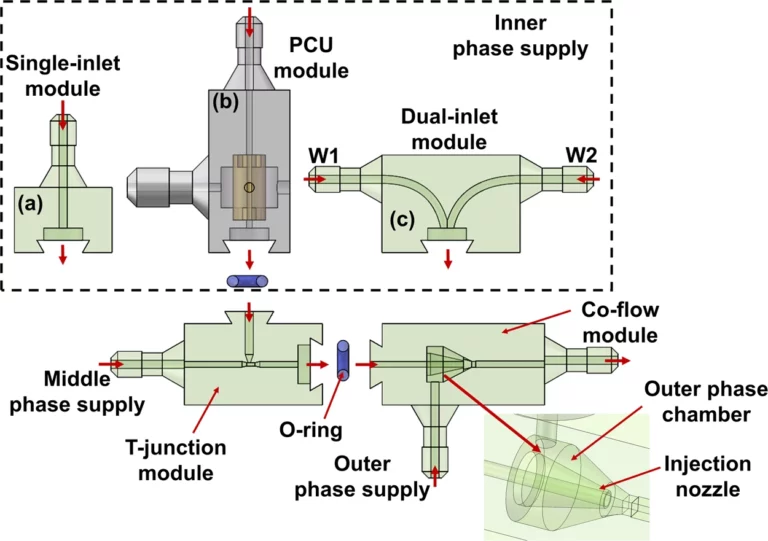
Conclusion
Recent advances in microfluidics have enabled the controlled production of monodispersed tunable multiple-emulsion droplets. However, double emulsions, water-in-oil-in-water, and oil-in-water-in-oil require complex spatially defined wettability control. PDMS surface wettability can be achieved using coating and non-coating techniques.
3D-printed microfluidics integrating a pressure-driven flow controller allows us to overcome the issues associated with these techniques.
Furthermore, 3D-printed technologies can be applied in other fields, such as material engineering and bioengineering. In the future, we believe that more complicated devices for microfluidics and more controllable methods for emulsion generation can be developed based on the rapidly growing 3D-printed technologies.
Â
Review written by Noémi Thomazo, PhD
Microfluidic research engineer
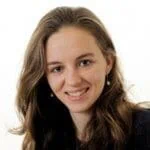
References
- Wang, N., et al., Modelling double emulsion formation in planar flow-focusing microchannels. Journal of Fluid Mechanics, 2020. 895: p. A22.
- Lu, P., L. Wu, and X. Liu, Visualization Study of Oil-in-Water-in-Oil (O/W/O) Double Emulsion Formation in a Simple and Robust Co-Flowing Microfluidic Device. Micromachines, 2017. 8(9): p. 268.
- Panizza, P., et al., Controlled production of hierarchically organized large emulsions and particles using assemblies on line of co-axial flow devices. Colloids and Surfaces A: Physicochemical and Engineering Aspects, 2008. 312(1): p. 24-31.
- Okushima, S., et al., Controlled production of monodisperse double emulsions by two-step droplet breakup in microfluidic devices. Langmuir, 2004. 20(23): p. 9905-9908.
- Nabavi, S.A., G.T. Vladisavljević, and V. Manović, Mechanisms and control of single-step microfluidic generation of multi-core double emulsion droplets. Chemical Engineering Journal, 2017. 322: p. 140-148.
- Zhu, P. and L. Wang, Passive and active droplet generation with microfluidics: a review. Lab on a Chip, 2017. 17(1): p. 34-75.
- Choe, G., et al., Hydrogel Biomaterials for Stem Cell Microencapsulation. Polymers, 2018. 10: p. 997.
- Männel, M.J., et al., Combining Hydrophilic and Hydrophobic Materials in 3D Printing for Fabricating Microfluidic Devices with Spatial Wettability. Advanced Materials Technologies, 2021. 6(9): p. 2100094.
- Trantidou, T., et al., Hydrophilic surface modification of PDMS for droplet microfluidics using a simple, quick, and robust method via PVA deposition. Microsystems & Nanoengineering, 2017. 3(1): p. 16091.
- Meissner, M., A.M. Seddon, and C.P. Royall, Chapter 6 – Colloidal Microfluidics, in Frontiers of Nanoscience, D. Chakrabarti and S. Sacanna, Editors. 2019, Elsevier. p. 125-166.
- Hu, S., et al., Surface-directed, graft polymerization within microfluidic channels. Anal Chem, 2004. 76(7): p. 1865-70.
- Abate, A.R., et al., Photoreactive coating for high-contrast spatial patterning of microfluidic device wettability. Lab Chip, 2008. 8(12): p. 2157-60.
- Bauer, W.-A.C., et al., Hydrophilic PDMS microchannels for high-throughput formation of oil-in-water microdroplets and water-in-oil-in-water double emulsions. Lab on a Chip, 2010. 10(14): p. 1814-1819.
- Kim, S.C., D.J. Sukovich, and A.R. Abate, Patterning microfluidic device wettability with spatially-controlled plasma oxidation. Lab on a Chip, 2015. 15(15): p. 3163-3169.
- Li, S., et al., Rapid preparation of highly reliable PDMS double emulsion microfluidic devices. RSC Advances, 2016. 6(31): p. 25927-25933.
- Su, R., F. Wang, and M.C. McAlpine, 3D printed microfluidics: advances in strategies, integration, and applications. Lab on a Chip, 2023. 23(5): p. 1279-1299.
- Ji, Q., et al., A Modular Microfluidic Device via Multimaterial 3D Printing for Emulsion Generation. Scientific Reports, 2018. 8(1): p. 4791.